Making an assumption is inevitable and reasonable in many research projects since it is practically impossible to handle all physics we know in a single study; even if it is feasible, it would rather squander resources, which are given to us. However, it is always required to evaluate carefully whether the assumptions are applicable for the particular project. Otherwise, the results may not be able to convince the community, even when the causal connection is well proven to be true.
In this post, I will introduce my recent work talking about one assumption people used to make for the study of Sagittarius A*: radiative cooling can be ignored.
The supermassive black hole in the Galactic Centre, Sagittarius A* (Sgr A∗), the only black hole for which we can directly resolve both the event horizon as well as its outer feeding conditions, thus it serves as a unique laboratory for numerical models of accretion and outflows. It is known to be fed by a radiatively inefficient accretion flow (RIAF), inferred by its low accretion rates. It is widely believed that, in this range of low accretion rates, the cooling time of electrons is much longer than the time required for them to fall into the black hole. Consequently, radiative cooling losses have, in general, been overlooked in the numerical study of Sgr A∗. However, the radiative properties and the non-linear nature of the plasma in RIAFs are poorly understood. For instance, how radiative cooling losses affect the turbulence features of the disk, and thus the angular momentum transport of the accreting plasma? How does radiative cooling together with the effects of general relativity result in the observed spectra from Sgr A*?
In this work, using full 3D general-relativistic magneto-hydrodynamical (GRMHD) simulations, we study the impact of radiative cooling on the dynamical evolution of the accreting plasma, presenting spectral energy distributions and synthetic sub-millimeter images generated from the accretion flow around Sgr A∗. These simulations calculate the approximated equations for radiative cooling processes self-consistently, including synchrotron, bremsstrahlung, and inverse Compton processes. Bremsstrahlung is relatively weak, while synchrotron and inverse Compton scattering are dominant up to 10 Rg, where Rg is the gravitational radius.
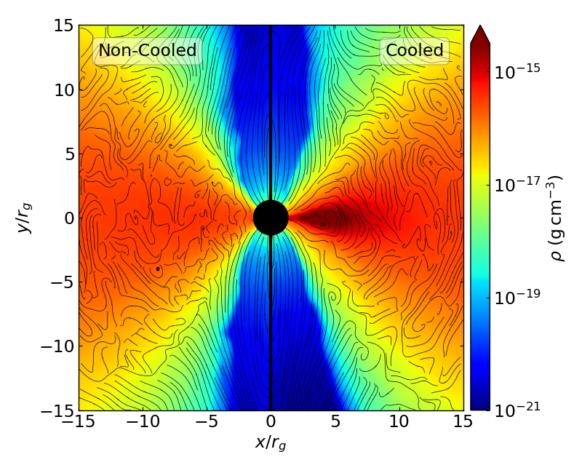
We find that radiative cooling plays an increasingly important role in the dynamics of the accretion flow as the accretion rate increases: the mid-plane density grows and the infalling gas is less turbulent as cooling becomes stronger (Fig 1). The changes in the dynamical evolution become important when the accretion rate is larger than 10^-8 Msun yr^-1. Since the measured accretion rate of Sgr A* lies within 10^-9 ~ 10^-7 Msun yr^-1, it shows that radiative cooling can, at least marginally, play a role in re-shaping the structure near the black hole.
It is believed that magneto-rotational instability (MRI) is the dominant mechanism for getting rid of the angular momentum in the form of a disk that has a low accretion rate and thus leading the materials to reach the black hole. What we can see is that radiative cooling reduces MRI-driven turbulences, impeding the gas inflow.
The resulting image at 230 GHz and spectra in the cooled model also differ from that in the non-cooled model: the intensity at the brightest region in the cooled model is slightly dimmer than in the non-cooled model. The overall flux including the peak values at the sub-mm and the far-UV is also slightly lower as a consequence of a decrease in the electron temperature. While the difference is not significant, our results suggest that radiative cooling needs to be taken into account in modeling Sgr A*.
Theoretical understanding of black hole astrophysics is very timely since the upcoming interferometric observation of Sgr A* with the Event Horizon Telescope (EHT) will enable us to stand on another breakthrough, imaging the black hole shadow around Sgr A*. Our study challenges the general assumption that radiative cooling can be ignored, and hope to contribute to predicting the images and understanding the physics of the accreting flows around Sgr A* in the right direction.