The Event Horizon Telescope (EHT), a ‘virtual’ Earth-sized telescope made by linking facilities across the globe, is on the cusp of announcing its first ever results to “photograph” a black hole!
Why does this relate to our research group? Well, we develop theoretical models for the physics of what happens to stuff as it falls into a black hole (and in some cases also escapes the pull and gets ejected in big streams of hot plasma). To know if a model is correct we need to be able to compare it to real data; i.e we use our model to make predictions for the light created just outside the black hole, and compare those predictions to what EHT and other telescopes meaure. Compared to all others telescopes EHT is special because it is the only one that has good enough resolution to actually see the black hole clearly (we call this “resolving the event horizon”).
We can’t actually see the black hole itself (by definition) but we CAN see the light coming from the surrounding material, which can lead to a donut-y sort of shape as the black hole “subtracts” light that falls within its Event Horizon (see images below). Many groups are actively working on making predictions of what exactly this will look like, which is made complicated by the extreme physical conditions and strong gravity that distorts the very fabric of spacetime. We have all developed our own models, that are written up into codes we run on large computer clusters/supercomputers, in order to make predictions. Our group uses a new code called HAMR that was developed by Matthew Liska when he was a MSc student in my group, and now as a PhD student in Michiel van der Klis’ group. Because this code is accelerated using GPUs (the same technique that makes video games work so fast), we can start to implement increasingly more realistic physical processes into our modeling, including keeping better track of the light production.
Most people don’t think about light as a carrier of energy, but that’s exactly what happens when you see something: light takes energy away from the system that emits it, and in doing so leaves the system cooler than it was. The same is true for stuff around black holes, and this cooling can actually change the structure of the flow around the black hole, which in turn changes how the system looks.
I looked at this problem around five years ago, together with two of my former PhD students (Dr. Samia Drappeau and Dr. Salomé Dibi), along with collaborators Prof. Chris Fragile (College of Charleston, USA) and Dr. Jason Dexter (MPA) (see here and here for the papers if you’re curious). We found that including this cooling in simulations of matter around black holes does in fact result in different predictions for the light coming out, but we did not go so far as to try to make actual images, a necessary step to compare to data from the EHT.
Now one of the MSc students in the group, David van Eijnatten, has been revisiting this problem with the new capabilities allowed by the HAMR code. He first implemented the cooling processes (which are only valid for very weakly accreting black holes such as the EHT targets, it should be noted), and ran simulations of a black hole with mass and accretion rate set to match that of Sgr A*, the supermassive black hole in the center of our own Milky Way Galaxy. Other measurements limit the accretion rate to fall in a rather narrow range, and our work suggests that even within this range, including cooling can affect the dynamics:
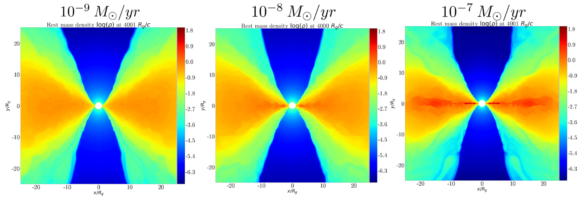
The difference between cooling and no cooling can have a big impact on what EHT will see. Here we show some predicted “ideal” images (i.e. not yet smeared out to the resolution of the telescope):
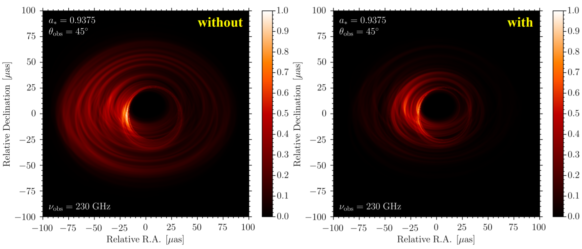
This is very early days for us, I would like to note that several other groups have been working on this type of calculation for longer! However we are the first to include radiation self-consistently and at such high resolution, so as we get further in this research we can compare our results to those from other groups, as an important contribution to the interpretation of EHT data.
If you think this is cool (get it?), please check out our SXSW Panel Picker pitch and vote for us (you will need to set up a free panelpicker account first)!!